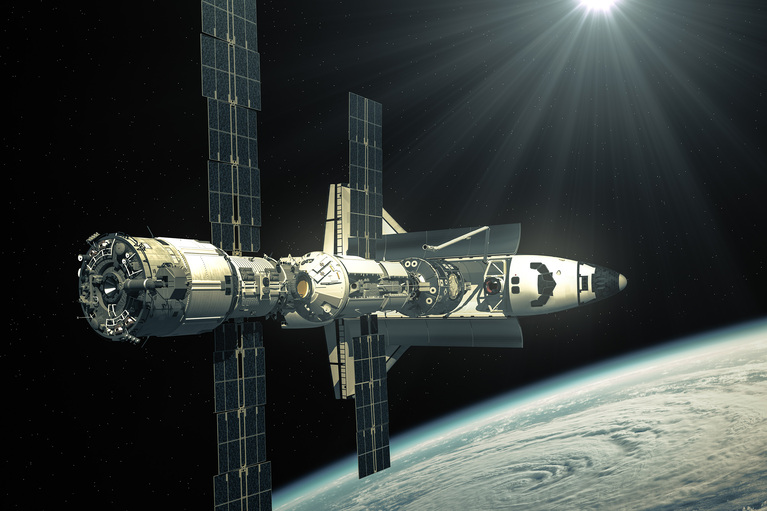
For New Space satellites, radiation tolerance means new design hurdle
Vicor power modules are power dense, reliable and scalable. Furthermore, there are multiple benefits of soft switching in radiation-tolerant power systems
This article is intended to provide a high level view of the fundamental environmental and design challenges associated with developing a power delivery network for space based applications like LEO and MEO communication satellites.
By Joe Dussi, Senior Regional Marketing Manager
This article will provide a general overview of some of the fundamental challenges and design considerations needed to design and manufacture a power supply capable of surviving in the harsh environment of space while reliably delivering power. These are very complex systems that require a high level of power system design expertise to ensure flawless operation.
The term “power delivery network” (PDN) is used to describe all aspects of power generation, storage, conditioning, distribution and conversion for all types of space-based applications (Figure 1). Power is ubiquitous across all space missions, which range in length of mission and purpose. For instance, launchers require power for just a few minutes during takeoff, while satellites, deep-space probes and orbital space stations need uninterrupted power for years and even decades. The power levels can range from a few watts to tens of kilowatts depending on the size and mission objective. Because of the extreme variation in power requirements, finding the optimum combination of primary and secondary power sources is the top priority of power systems engineering.
Figure 1: Example of typical circuit block diagram for a satellite power delivery network.
To adequately meet the requirements of the mission there are a number of power sources that can be combined to achieve the most efficient solution. Today, most satellites rely on advanced solar cells with an efficiency around 30% and on Li-ion batteries. Nuclear power is also a viable option, but this is only necessary beyond the effective range of the Sun’s solar rays, which is roughly beyond Jupiter.
In space size and weight are critical design parameters that require close attention in a power system design. In many cases the power delivery network represents a significant percentage of the weight of the satellite (Figure 2), and because of that there are constant design efforts to reduce the weight and size of the solution while simultaneously improving the power output.
Figure 2: NASA-sourced photo showing Hubble Telescope battery pack assembly.
You may ask, how do I shrink the power supply and get more usable power? This is a bit of a design conundrum. With advancements in solar cell and battery technologies, power design architects have been able to close that gap. Another way to maximize your available power budget is to use the latest advanced power electronics circuitry. Factors like power density and efficiency become very important when designing a satellite PDN. By increasing the power density and efficiency you are able to shrink the overall board space of the power circuitry and reduce the weight (Figure 3).
Figure 3: Displaying a Vicor high-density, high-efficiency modular power demo board for satellite.
Another benefit of having a higher-efficiency solution is reduction of thermal dissipation that needs to be accounted for in the design, meaning you can reduce the size of or maybe even eliminate the need for a heat sink that would otherwise add weight and space to the design. Since there is no air or wind in space, the heat transfer must occur through “conduction,” meaning the contact surface should be as uniform as possible to maximize the effective contact area (Figure 4). Advancements in semiconductor materials such as Gallium Nitride (GaN) or Silicon Carbide (SiC) are also helping power designers to improve overall power system performance.
Figure 4: 3D rendering of Vicor space demo board showing heat spreader hovering over power modules.
As demand for internet bandwidth continues to soar around the world, satellite communications providers and OEMs are striving to develop products to take advantage of this explosive growth opportunity. Satellite communications providers and OEMs are planning to deploy enough capacity to increase overall bandwidth by at least 20x over the next five years (Figure 5). To accomplish this, the industry is undergoing a massive paradigm shift in how they design satellite systems. A key element of the overall problem is solving the power challenge. New Space satellite communication solutions demand high-performance processing, which in turn requires more power. Limited space and weight budgets therefore put a premium on advanced power solutions with high density and high efficiency to enable these advanced communications systems.
Figure 5: Explosive growth in the commercialization of space.
These new LEO and MEO satellite constellations represent billions of dollars in investment. Therefore, a reliable, uninterrupted supply of power is essential to a space mission's success. In space the primary source of energy is the Sun, which provides approximately 1.4 kilowatts of power per square meter in Earth orbit. This is why the majority of spacecraft incorporate large solar arrays to harness the power of the Sun’s solar rays (Figure 6). These are composed of an interconnected network of photovoltaic cells, which produce an electrical current when light shines on them.
Figure 6: Solar PV panels deployed on a space station in orbit to capture the sun’s solar rays to convert to energy.
Even with all this free and readily available power, designing a space-based power supply can be a daunting design challenge. Today’s photovoltaic cells have efficiencies around 30%, but that efficiency rating is reduced by heating from the Sun and radiation damage during a satellite's lifetime. This means that solar arrays have to be of a significant size to deliver the necessary power levels.
To add to this complexity, that most satellites have orbits that will take them out of the Sun and into the shadow of Earth (Figure 7). This means that the satellite needs a secondary power source to bridge the solar gap. This secondary source is typically a rechargeable battery that keeps the satellite powered while in earth’s umbra.
Figure 7: Anticipated orbital path of a satellite as it rotates around the earth.
A satellite's electrical loads will often vary, depending on which instruments or subsystems are running at a particular time. One of the many tasks of the PDN is to control the exchanges of power between the solar generator, the battery and the loads. This means ensuring that the power delivered to the loads stays within the voltage ranges each point-of-load is designed for.
For New Space satellites, radiation tolerance means new design hurdle
Vicor power modules are power dense, reliable and scalable. Furthermore, there are multiple benefits of soft switching in radiation-tolerant power systems
60th Annual AOC International Symposium & Convention
The premier EW and Radar technology event of the year
Vicor power modules set new standards for satellite communications
Explore cutting edge power solutions at Space Tech Expo Europe
Powering change
Patrizio Vinciarelli, CEO of Vicor, quietly solves the toughest power challenges on the planet — automotive electrification and powering AI