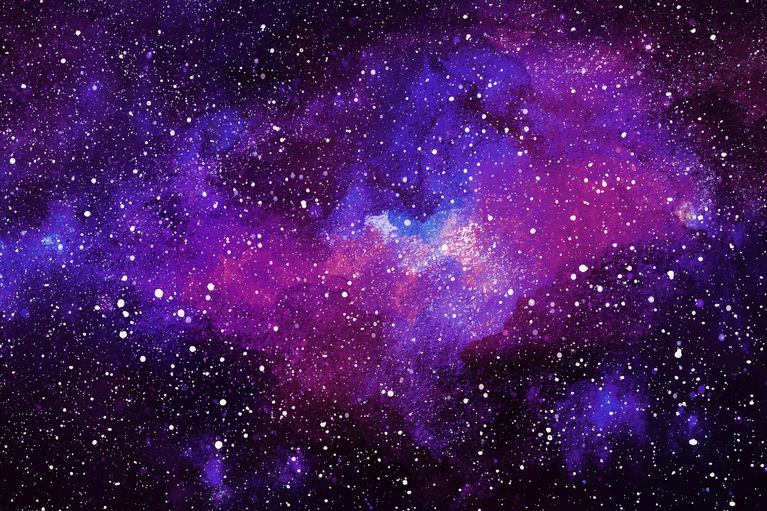
Microgate and Vicor partner to unlock deep space secrets
瞭解高密度電源模組如何幫助巨大的地球望遠鏡增强深空影像
White Paper by Maurizio Salato
AC-DC converters for industrial applications have been relying on double-stage architecture for quite a long time. A non-isolated, single- or three-phase, single- or multi-level stage typically feeds an isolated DC-DC stage, with capacitors between the two stages providing the necessary energy storage. On the other end, renewed interest in DC power systems drive new developments in the field of fixed-ratio bus converters, with very high performance to cost ratios. This paper proposes a new AC-DC system architecture based on fixed-ratio bus converters. System functions are described and analyzed for the classic as well as the proposed approach; benefits, trade-offs and design methodology for the novel architecture are also discussed.
The need for highest availability in the telecom systems world has driven the adoption of SELV DC levels of voltage for power distribution, with specialized architectural approaches. At the same time, the need for high efficiency and high density has determined the evolution of distributed power systems toward architectures that employ optimized power components. Among those power components, fixed-ratio bus converters have been developed to provide state-of-the-art performance. The need for high-power, high-density systems has also driven the development of equivalent, fixed-ratio bus converters capable of 300 – 400VDC input and isolated, Safety-Extra-Low-Voltage (SELV) output. This paper proposes a new approach in medium-power industrial rectifier systems, aiming at leveraging the availability of such power components, as shown in Figure 1.
Figure 1: Replacement of DC-DC stage in medium-power rectifiers with bus converters.
For the purpose of this analysis, single-phase and three-phase systems will be discussed separately.
Single-phase systems present the challenge of storing the necessary energy to sustain the DC load while the power drawn from the AC line follows its sinusoidal nature.
The established power system architecture is based on a two-stage approach as shown in Figure 2, where the systems functions are listed below each element. For the purpose of this paper, it is interesting to notice that:
where POUT and VOUT are the output power and voltage of the AC-DC stage, and k ≅ 0.8 – 0.9 is a constant.
The total capacitance installed in a classic rectifier system, as shown in Figure 2, is therefore determined by the ripple current capability of the chosen capacitors bank. This implies relatively large capacitors, which maintain a relatively low-line frequency ripple as well as voltage drop during hold up applied to the DC-DC stage.
Input: single phase AC; Output: 48V, 25A, 1200W; Hold-up time: 10ms, full load. Figure 3 shows the AC-DC output voltage ripple and the capacitor’s RMS current capability for the considered case with general purpose 450V Al-electrolytic capacitors.
Figure 2: Classic rectifier system elements.
Figure 3: 1200W AC-DC stage line frequency RMS ripple voltage on 390VDC output and 450V Al electrolytic capacitor current ripple capability vs. capacitance (Nippon Chemi‑Con KMT Series)
A 1mF, 450V Al-electrolytic capacitor, rated to sustain ~5ARMS 120Hz current ripple, can easily fit this application, which requires ~3ARMS at full load; with this configuration, the DC-DC stage needs to reject ~14VPK-PK line frequency voltage ripple at full load, while during hold-up time the capacitor will discharge from 390 to 358V, which corresponds to a mere 16% of the total energy stored. System efficiency of 94% can be achieved, with the DC-DC converter stage accounting for 60 – 70% of total power losses (~96 – 97% efficient). Equations 2 and 3 show the approximate system efficiency as well as a baseline of system volume breakdown.
Following are two notable issues with this standard architecture:
The proposed architecture is based on the consideration that system operations in regular conditions do not require a regulated second stage, as the AC-DC stage can easily accomplish average, “bulk-type” regulation. A bus converter can be implemented, with an auxiliary “ripple compensation stage”, as shown in Figure 4.
Figure 4: Proposed rectifier system with bus converter and auxiliary DC-DC converter
The bus converter now steps down (or up) the output of the AC-DC stage, while providing isolation. Stacked with its output, a small isolated DC-DC converter stage actively compensates for the line frequency ripple that is simply transferred through (and scaled down by) the bus converter. Considerations regarding installed capacitance still apply, however the AC-DC stage is now regulating the load most of the time. Bus converters are typically 1.5 – 5% higher efficiency and 2 – 3x denser than DC-DC converters; because majority of power (>85%) is now processed by the bus converter stage, significant density and efficiency improvements are obtained at system level.
Input: single-phase AC; Output: 48V, 25A, 1200W; 1mF, 450V Al-electrolytic capacitor.
During hold up, the capacitor experiences a minimum potential difference of 358V, at which point the DC-DC auxiliary converter will need to provide up to 48 – 358V/8 = 3.5V, which translates to less than 90W at 25A output. Because the DC-DC stage only processes 2 – 4% of overall power, even a relatively inefficient design would not affect the overall result, mostly determined by the bus converter performance. Equations 4 and 5 show the estimated total system efficiency as well as the system volume breakdown:
Because bus converters are bidirectional in nature and have the characteristic of broadband transformers, energy storage can be placed either at the input (high voltage) or output (low voltage), like for example shown in Figure 5. This option provides the designers with choices previously unavailable for energy storage other than capacitors, such as ultra-capacitors or high-density batteries.
Figure 5: Secondary side energy storage option with specialized storage elements
Moreover, secondary-side energy storage avoids propagation of input line transients to the storage elements, which in turns increases their overall lifetime and reduces the need for significant de-rating.
Several different approaches have been used in the past decades for active three-phase rectification. The main advantage of several converter topologies for three-phase PFCs is the use of storage elements for filtering purposes rather than line-frequency energy storage. The simplest explanation is that single phase AC-DC converters face the challenge of storing the necessary energy to sustain the DC load while the power drawn from the AC line follows its sinusoidal nature; this implies a steady, low frequency current flow through the storage elements. Three-phase AC systems can instead provide continuous power flow in regular operations and functional filtering can be ideally limited to the converter switching current components.
Where a single storage element is utilized within the converter (an electrolytic capacitor). Figure 6 shows how available storage components (capacitors and batteries) fit the requirements of the power system as function of energy storage density and electrical time constant required by the application. As stated before, AC line ripple filtering can be greatly reduced in this case; at the same time, more and more systems don’t require hold up anymore, but rather short term back up (typically up to 1 min, after which generator sets guarantee the energy supply). To the rectifier system design, this market trend translates into the possibility of avoiding medium density storage elements altogether.
The only standing issue with respect to medium density, medium speed storage elimination is the phase-loss event. Losing one phase in a three-phase rectifier system requires the filter capacitors to provide the line-frequency AC current that would have been provided by the converter branch normally powered by the missing phase. If a short term back-up is required, the converter can actually be managed very simply and effectively by equating the phase-loss event to a complete line brown-out: converter should stop operating and rely on downstream batteries or ultra-capacitors.
Figure 6: Electrical energy storage elements space – application required electrical time constant vs. energy storage density.
Input: three-phase, three-wire 400 – 480VAC; Output: 48V, 150A, 7200W, with secondary-side battery back up.
Classic rectifier architecture is shown in Figure 7, while the proposed one, based on bus conversion, is shown in Figure 8. Beside the DC-DC converter stage being replaced with a bus converter, the capacitors between the two stages have also been changed from electrolytic to non-polarized, film‑polymer type, useful for switching noise filtering rather than line-frequency energy storage.
Figure 7: Traditional telecom rectifier architecture diagram.
Figure 8: New telecom rectifier architecture enabled by bus converter.
The application-specific characteristics of interest between a regulated DC-DC stage and a bus converter are listed in the following table:
Power Flow
Bandwidth
Regulation
Input to output characteristic
The bus converter presents the unique opportunity to leverage an existing, well established battery back-up system as line frequency filter element, reducing (or possibly completely eliminating) the need for storage components such as electrolytic capacitors. To better understand how this works, it is useful to refer to AC models of both the bus converter (shown in Figure 9) and generic lead-acid batteries (still widely diffused, although the same approach would be applicable with advanced battery technologies, such as NiMH or Li-ion).
Figure 9: Bus converter AC model diagram.
Figure 10: Lead-acid battery third-order model diagram.
Figure 11: Bus converter and lead-acid battery low-frequency (<1kHz) AC model diagram.
Figure 12: AC model of Figure 11 with primary-referenced parameters diagram.
Table 2 summarizes the modeling parameters and their value range and an example of parameters for AC models is shown in Figures 11 and 12.
ROUT
n
R1
R2
C1
The broadband, bidirectional characteristic of the bus converter enables the battery to act as bulk component with respect to the AC-DC stage. The frequency characteristic of the battery, as “seen” from the AC-DC converter, will therefore be a simple first-order low-pass filter with slightly variable gain (and variable cut-off frequency); using Table 2's range of values, its time constant ranges between 1000 and 10000s, effectively acting as a very large capacitor with respect to the rectifier first stage. It is useful to remember that, while large batteries might be able to support operations with a missing phase (because of relatively low internal resistance), it could be conceivable to leverage the battery backup during any anomaly on the input AC line: missing phase, brownout or voltage sags. Line transients wouldn’t affect the small, non-polarized capacitance between the two stages while the output would be completely decoupled.
Bus converters can be used to enhance density and efficiency figures of industrial rectifier systems. They enable flexibility regarding local energy storage, which can be either placed on the high-voltage side or the low-voltage side, or both. For medium and large systems, the bus converter enables the use of existing (or otherwise necessary) battery backup on the low-voltage output as bulk power storage element for power conversion purposes, eliminating the need for large (and potentially unreliable) electrolytic capacitors.